Neural Circuits Generating the Rhythm of Walking: Latest Insights into the Central Pattern Generator (CPG)
The act of "walking," something we usually do without conscious thought, is actually controlled by a highly sophisticated neural mechanism. The Central Pattern Generator (CPG), a neural network, plays a central role in this process. The CPG has the ability to autonomously generate rhythmic motor patterns, such as walking, even without commands from the brain or sensory information. This article explains the basic mechanisms of the walking CPG, mainly thought to reside in the spinal cord, along with new findings revealed by recent research and its applications in rehabilitation.
What is a CPG?
A CPG is a network of neurons within the spinal cord (or brainstem) that can generate periodic motor commands without continuous input from the brain or sensory feedback from the periphery [1]. In walking, it forms the basis for coordinated movements of the left and right legs, as well as alternating activity patterns of flexor muscles (muscles that bend the leg) and extensor muscles (muscles that straighten the leg). This fundamental rhythm-generating ability allows us to walk smoothly without conscious effort.
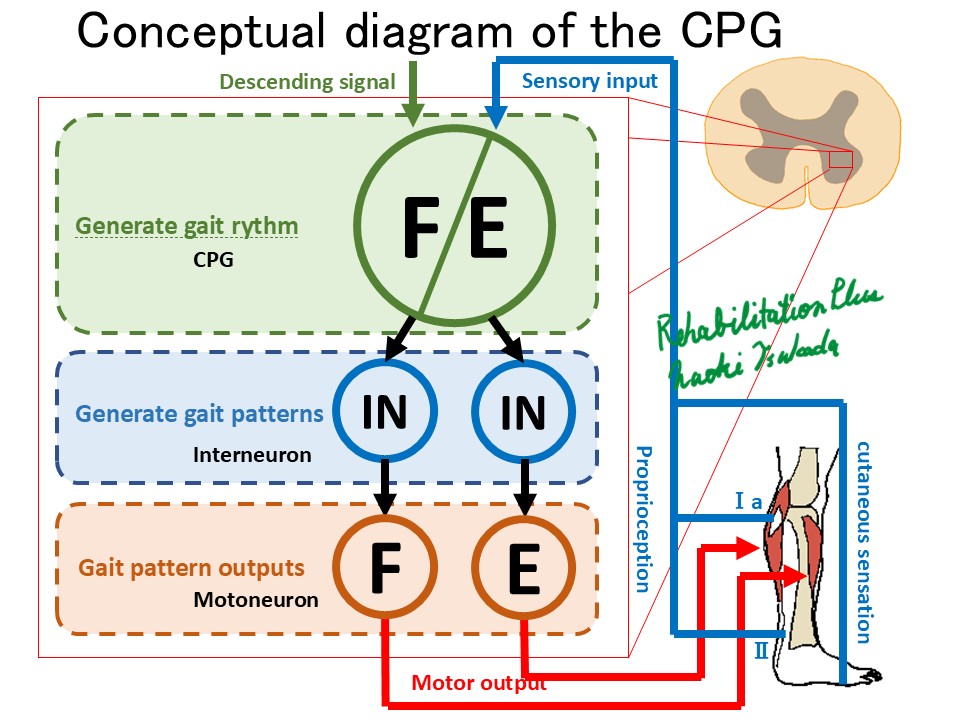
Basic Mechanisms of CPGs and Recent Advances
The existence of CPGs was suggested in the past through experiments using decerebrate cats. It was observed that even when the spinal cord was disconnected from the brain, walking-like movements could be induced if the cat was placed on a treadmill with appropriate sensory input.
The core of the CPG is believed to be a complex network of interneurons in the spinal cord. These neurons are interconnected and exchange excitatory and inhibitory signals, generating rhythmic firing patterns, which constitute the rhythm of walking. Recent research using genetic techniques and optogenetics is advancing the identification of specific interneuron groups that make up the CPG and the elucidation of their functions [2]. For example, developmentally classified neuron groups such as V0, V1, V2a, and V3 have been shown in animal models like mice to play specific roles in left-right coordination, alternating flexor-extensor activity, and the stability of rhythm generation, respectively [1, 3]. The detailed circuit mechanisms of how these neuron groups interact to create a stable walking rhythm are being actively investigated.
How is CPG Activity Regulated?
While CPGs can generate rhythms autonomously, actual walking is skillfully adjusted according to the situation. Two main factors are involved in this adjustment:
Sensory Feedback
Peripheral sensory information, such as ground contact sensation from the soles of the feet and proprioceptive sensation (the sense of the position and movement of limbs) from muscles and tendons, is input to the spinal CPG in real time. This allows for fine-tuning of the walking pattern to respond to changes in the ground surface or to quickly regain posture when stumbling (stumble recovery reflex) [3, 4]. Recent studies are gradually clarifying the specific pathways through which certain sensory inputs act on which neuron groups within the CPG and how they modulate the walking rhythm [4].
Descending Control from the Brain
Intentional control of starting and stopping walking, changing speed, turning direction, or avoiding obstacles is performed by commands from the brain. In particular, the Mesencephalic Locomotor Region (MLR) in the brainstem is known to play an important role in initiating walking and regulating its speed [5]. Signals from the MLR activate the spinal CPG network, and the walking speed changes according to the level of this activity. The cerebral cortex and cerebellum are also involved in higher-level walking planning and balance control, precisely adjusting CPG activity. Recent research suggests that these descending pathways may selectively control specific interneuron groups that make up the CPG, thereby enabling diverse walking patterns [5].
Applications of CPGs in Rehabilitation
The concept of CPGs is particularly important in rehabilitation after spinal cord injury (SCI). Even if descending commands from the brain are severed due to SCI, the CPG network itself, located in the spinal cord below the injury site, may remain intact. Therefore, if the CPG can be appropriately activated, it may be possible to partially recover lost walking function.
Traditional rehabilitation includes Body Weight Supported Treadmill Training (BWSTT). This involves walking on a treadmill with partial body weight support and aims to activate the spinal CPG through appropriate stimulation of the soles of the feet and sensory feedback from hip extension.
Furthermore, in recent years, remarkable progress has been made in the development of Epidural Electrical Stimulation (EES) as a treatment [6, 7]. This involves implanting electrodes in the epidural space of the spinal cord and continuously or periodically stimulating the lumbosacral region where the CPG is thought to reside. Recent research has shown that providing spatiotemporally patterned stimulation synchronized with the walking cycle, rather than simply stimulating, can induce more natural and functional walking movements [6, 7]. In some patients with complete paralysis, combining EES with intensive rehabilitation has resulted in the ability to perform limited voluntary walking even without stimulation, demonstrating the new potential of neurorehabilitation targeting CPGs [7]. In the future, the development of more advanced treatments that combine EES with Brain-Machine Interfaces (BMIs) to control stimulation patterns according to the patient's intention is expected.
It is important to consider various aspects, but here are some ideas based on the content of this article:
Focusing on Descending Signals
It is important to correct head tilt and body alignment, minimizing the sensation of leaning or falling as much as possible. Devices such as BWSTT or slings may be effective at times.
Walking slowly increases the stance time on one leg, making it less likely for inertia to occur. Even healthy adults can become unsteady when walking slowly. Therefore, it can sometimes be useful to have two people assist with walking to experience speed and rhythm.
Focusing on Sensory Feedback
When focusing on sensory feedback, the feedback from the lower limbs during weight-bearing is crucial. In particular, cutaneous sensation from the sole of the foot and proprioceptive sensation such as Ia and Ib information from the muscle spindle and Golgi tendon organ during stance are important for recognizing the support status of the leg.
It may be helpful to check whether the extensors on the stance side are inactive in a standing position by assessing the length of the extensors (Ia afferent information from muscle spindles) and the tension of the tendons (Ib afferent information from Golgi tendon organs).
What About Orthotics?
There are several issues with orthotics that fix the ankle joint. For example, after heel strike, the lower leg is pushed from behind, resulting in a sensory feedback of knee buckling. This information can potentially signal the CPG to stop walking.
However, by selecting appropriate situations, orthotics can facilitate rehabilitation, such as practicing overall anti-gravity extension during stance, and provide a sense of stability that allows for more walking experience.
Conclusion
The walking CPG is an autonomous rhythm-generating circuit located in the spinal cord and forms the basis of our fundamental walking movements. Its activity is skillfully adjusted by peripheral sensory feedback and descending commands from the brain. Recent research has deepened our understanding of the specific neuronal networks that constitute the CPG and their regulatory mechanisms. We believe these findings indicate the importance of a multifaceted approach in new rehabilitation strategies for walking disorders.
In conclusion, we have summarized the role of CPGs during walking. It is important to note that the CPG is only one aspect of walking, and gait training focusing solely on the CPG will not result in functional walking.
References
- Kiehn, O. (2016). Decoding the organization of spinal circuits that control locomotion. Nature Reviews Neuroscience, 17(4), 224-238. (※Slightly older, but a crucial review for understanding the foundation of CPG research and the basis for papers within the last 5 years)
- Gosgnach, S., Bikoff, J. B., Dougherty, K. J., El Manira, A., Lanuza, G. M., & Zhang, Y. (2017). V0 interneurons coordinate limbed locomotion with breathing. Neuron, 95(5), 1167-1178.e6. (※An example of elucidating the function of specific interneurons, although related to coordination with breathing)
- Danner, S. M., Zhang, H., Shevtsova, N. A., & Rybak, I. A. (2019). State-dependent signaling in spinal cord locomotor command pathways. Current Opinion in Physiology, 8, 82-90.
- Takeoka, A., & Arber, S. (2019). Functional Local Proprioceptive Input to Motor Pools Formed by Renshaw Cell Neuromodulation. Cell, 177(3), 749-763.e18.
- Jordan, L. M., & Rybak, I. A. (2022). Neural Control of Locomotion: Systems, Circuits, and Neurorehabilitation. Comprehensive Physiology, 12(1), 3061-3137. (※A comprehensive review that includes recent findings on descending control such as MLR)
- Wagner, F. B., Mignardot, J. B., Le Goff-Mignardot, C. G., Demesmaeker, R., Komi, S., Capogrosso, M., ... & Courtine, G. (2018). Targeted neurotechnology restores walking in humans with spinal cord injury. Nature, 563(7729), 65-71.
- Rowald, A., Komi, S., Demesmaeker, R., Baaklini, E., Hernandez-Charpak, S. D., Paoles, E., ... & Courtine, G. (2022). Activity-dependent spinal cord neuromodulation rapidly restores trunk and leg motor functions after complete paralysis. Nature Medicine, 28(2), 260-271.